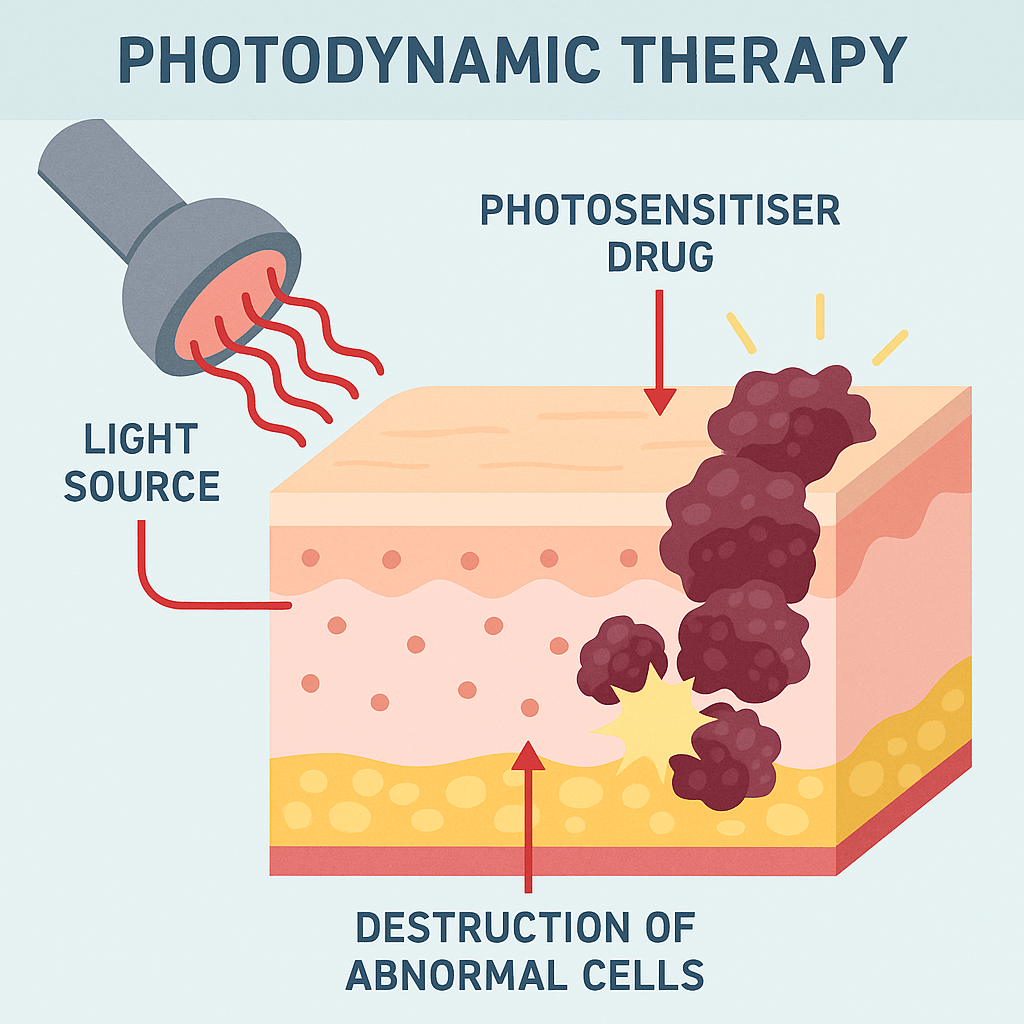
Introduction: Shining a Light on Skin Cancer Treatment
Skin cancer represents a significant global health concern, with its prevalence continuing to rise. This necessitates the ongoing development and refinement of diverse treatment options to effectively manage the disease while minimising morbidity. Among these advancements is Photodynamic Therapy (PDT), a targeted and relatively non-invasive approach that harnesses the power of light and specific chemical agents to selectively destroy cancerous cells.
At its core, PDT involves the application of a photosensitising cream followed by exposure to a particular wavelength of light, initiating a cascade of events that leads to the demise of malignant tissue. This article will delve into the intricate scientific mechanisms underpinning PDT, elucidating how photosensitising agents and precisely chosen light wavelengths work in concert to selectively target and eliminate skin cancer cells.
The Triad of PDT: Light, Photosensitizers, and Oxygen
The efficacy of Photodynamic Therapy hinges on the synergistic interaction of three key components: a photosensitising agent, a specific wavelength of light, and molecular oxygen.
A. Photosensitising Agents: The Key to Selectivity
Photosensitisers are chemical compounds that exhibit the unique property of becoming activated when exposed to light of a specific wavelength. In the context of dermatological PDT for skin cancer, these agents are typically administered topically, applied directly to the affected skin. A crucial characteristic of effective photosensitisers is their preferential accumulation within cancerous tissues compared to surrounding healthy cells. This selective uptake is paramount to PDT’s targeted action, minimising damage to healthy skin. Commonly employed photosensitisers in dermatology include aminolevulinic acid (ALA) and methyl aminolevulinate (MAL).
These compounds are, in fact, prodrugs, meaning they are precursors that undergo metabolic conversion within the skin cells to form the active photosensitiser, protoporphyrin IX (PpIX). This intracellular conversion process further contributes to the selective accumulation of the active agent within the target cancerous cells, enhancing the precision of the treatment.
B. Specific Light Wavelengths: Activating the Agent
Light, as part of the electromagnetic spectrum, encompasses a broad range of wavelengths, only a small portion of which is visible to the human eye. Photosensitisers are designed to absorb light within specific regions of this spectrum. The efficiency with which a photosensitiser is activated is highly dependent on the wavelength of light it is exposed to, with maximal activation occurring at its peak absorption wavelength.
In dermatological PDT, the light source employed must be carefully matched to the absorption spectrum of the photosensitiser being used. Various light sources are utilised, including light-emitting diodes (LEDs) and lasers, each capable of emitting light at specific and controlled wavelengths. For instance, protoporphyrin IX (PpIX), the active photosensitiser derived from ALA and MAL, exhibits strong absorption in the red light spectrum (around 630 nm).
Furthermore, the wavelength of light also dictates its penetration depth into the skin tissue. Longer wavelengths, such as red light, can penetrate deeper than shorter wavelengths like blue or green light. This characteristic is a critical consideration when treating different types and depths of skin cancer. Superficial lesions may be effectively treated with shorter wavelengths, while deeper-seated tumours may necessitate the use of longer wavelengths to ensure adequate activation of the photosensitiser throughout the cancerous tissue.
C. Oxygen’s Crucial Role: The Cytotoxic Punch
The activation of the photosensitiser by the appropriate wavelength of light is only the first step in the therapeutic process. The subsequent crucial element is the presence of molecular oxygen within the tissue. When the light-activated photosensitiser transfers its energy to surrounding oxygen molecules, it leads to the formation of highly reactive oxygen species (ROS), most notably singlet oxygen (1O2).
These ROS are potent cytotoxic agents, meaning they are highly damaging to cells. Their primary mechanism of action involves the rapid oxidation of essential cellular components, including lipids, proteins, and nucleic acids. The short lifespan and limited diffusion distance of singlet oxygen ensure that its destructive effects are largely confined to the immediate vicinity of the activated photosensitiser, thereby contributing significantly to the targeted destruction of the cancer cells that have accumulated the photosensitiser. This localised action is a key factor in PDT’s ability to selectively eradicate cancerous tissue while sparing adjacent healthy structures.
The Cellular and Molecular Mechanisms of PDT-Induced Cell Death
The generation of reactive oxygen species within the photosensitised cancer cells initiates a cascade of destructive events at the cellular and molecular levels, ultimately leading to cell death. PDT’s efficacy stems from its ability to inflict damage through multiple interconnected pathways.
A. Direct Cell Damage:
The highly reactive nature of singlet oxygen and other ROS produced during PDT results in significant oxidative damage to essential cellular components. This direct damage plays a pivotal role in triggering cell death:
- Lipid Peroxidation: ROS can attack the polyunsaturated fatty acid chains within cellular membranes, initiating a chain reaction known as lipid peroxidation. This process disrupts the integrity and function of cell membranes, leading to increased permeability and eventual cellular lysis.
- Protein Oxidation: Essential proteins within the cell are also susceptible to oxidation by ROS. This can lead to the denaturation and inactivation of critical enzymes involved in cellular metabolism, as well as damage to structural proteins that maintain cell shape and integrity.
- DNA Damage: The genetic material of the cell is another key target of ROS. Oxidative damage to DNA can result in strand breaks and base modifications, leading to genetic instability and the triggering of cell death pathways.
- Induction of Apoptosis: PDT can activate various signalling pathways within the cancer cells that lead to apoptosis, or programmed cell death. This is a controlled and regulated process characterised by specific morphological and biochemical changes, ultimately resulting in the orderly dismantling of the cell without causing significant inflammation.
- Potential for Necrosis: In cases of severe and rapid oxidative stress, PDT can also induce necrosis, a form of uncontrolled cell death characterised by cellular swelling and lysis, which can release inflammatory mediators into the surrounding tissue.
B. Vascular Damage:
Beyond directly targeting cancer cells, PDT also exerts a significant effect on the tumour microvasculature, the network of small blood vessels that supply nutrients and oxygen to the tumour:
- PDT’s effect on the tumour microvasculature: Photosensitisers can accumulate in the endothelial cells lining these blood vessels. Upon light activation, the generated ROS damages these cells.
- Damage to endothelial cells: This damage leads to a cascade of events, including vasoconstriction (narrowing of blood vessels) and increased vascular permeability.
- Vascular shutdown and nutrient deprivation: The resulting reduction in blood flow deprives the tumour of essential oxygen and nutrients, contributing to further tumour cell death and inhibiting its growth. This vascular-targeting effect is a crucial aspect of PDT’s overall anti-cancer activity.
C. Immune Response Modulation:
Emerging evidence suggests that PDT is not solely a local ablative therapy but can also modulate the host’s immune response to the tumour:
- How PDT can trigger a local and systemic anti-tumour immune response: The cellular damage induced by PDT can release various intracellular molecules into the tumour microenvironment.
- Release of damage-associated molecular patterns (DAMPs): These DAMPs act as danger signals, alerting the immune system to the presence of cellular stress and damage. They can bind to pattern recognition receptors on immune cells, leading to their activation.
- Recruitment of immune cells: The release of cytokines and chemokines following PDT can attract immune cells, such as dendritic cells (antigen-presenting cells) and T lymphocytes (cytotoxic and helper T cells), to the tumour site.
- Potential for long-term anti-tumour immunity: In some instances, the PDT-induced immune response can lead to the development of long-term anti-tumour immunity, potentially preventing local recurrence and even distant metastasis. This immunomodulatory aspect of PDT is an area of active research and holds significant promise for enhancing its therapeutic efficacy.
Factors Influencing PDT Efficacy in Skin Cancer Treatment
The effectiveness of Photodynamic Therapy in treating skin cancer is influenced by a multitude of factors, which can be broadly categorised as characteristics of the photosensitiser, the light source, the tumour itself, and the patient.
A. Photosensitiser Characteristics:
Several properties of the photosensitising agent significantly impact the outcome of PDT:
- Uptake and retention in tumour tissue: The degree to which the photosensitiser is selectively absorbed and retained by cancerous cells versus healthy tissue is paramount. Higher tumour-to-normal tissue ratios lead to more targeted destruction.
- Photochemical properties: The quantum yield (efficiency of singlet oxygen generation upon light absorption) and the specific absorption spectrum of the photosensitiser directly influence its ability to produce cytotoxic ROS when illuminated.
- Dark toxicity: An ideal photosensitiser exhibits minimal or no toxicity in the absence of light to prevent unwanted damage to healthy tissues.
B. Light Source Parameters:
The characteristics of the light source employed are equally critical:
- Wavelength and its match to the photosensitiser: As previously discussed, the wavelength of the light must closely match the absorption peak of the photosensitiser to ensure efficient activation.
- Light dose and irradiance: The total amount of light delivered (dose) and the rate at which it is delivered (irradiance) are crucial parameters that need to be carefully controlled to achieve optimal therapeutic effect without causing excessive damage.
- Penetration depth and treatment area: The wavelength of light influences its penetration depth into the tissue, which must be sufficient to reach the target tumour cells. The size and shape of the light delivery system must also be appropriate for the treatment area.
C. Tumour Characteristics:
The nature of the skin cancer being treated also plays a significant role in PDT’s success:
- Type and stage of skin cancer: PDT has demonstrated efficacy in treating various types of superficial skin cancers, such as basal cell carcinoma, squamous cell carcinoma in situ (Bowen’s disease), and actinic keratoses. However, its effectiveness may vary depending on the specific histological subtype and stage of the cancer.
- Thickness and size of the lesion: PDT is generally most effective for thinner and smaller lesions, as light penetration may be limited in thicker tumours.
- Vascularity of the tumour: The extent of blood vessel growth within the tumour can influence the delivery and activation of the photosensitiser and the subsequent vascular damage induced by PDT.
D. Patient Factors:
Individual patient characteristics can also affect the outcome of PDT:
- Skin type and sensitivity: Patients with different skin types may exhibit varying degrees of photosensitivity following PDT treatment.
- Overall health and immune status: The patient’s general health and the competence of their immune system can influence their response to PDT and their ability to clear the treated tissue.
Conclusion: Harnessing Light for Targeted Cancer Therapy
Photodynamic Therapy stands as a compelling and scientifically grounded modality for the treatment of skin cancer. By strategically employing photosensitising agents and specific wavelengths of light, PDT initiates a cascade of events that selectively target and destroy cancerous cells through direct cellular damage, vascular disruption, and the potential modulation of anti-tumour immune responses. Its advantages, including targeted action, the potential for excellent cosmetic outcomes with minimal scarring, and the possibility of repeat treatments, make it a valuable tool in the dermatological armamentarium.
Ongoing research continues to refine PDT protocols, explore new photosensitisers, and investigate its application in treating a broader range of dermatological conditions and even other forms of cancer, further underscoring the potent power of light in modern medicine.
Ready to Explore the Benefits of Photodynamic Therapy?
At the Skin Care Network, our experienced consultant dermatologists are at the forefront of innovative skin cancer treatments, including Photodynamic Therapy. If you’re seeking a targeted and less invasive approach for managing superficial skin cancer or precancerous lesions, PDT may be a suitable option for you.
Contact us today to schedule a consultation and discuss your individual needs.